Vol. 43 No.2 - Highlights
Towards high-temperature superconductors (Vol. 43 No. 2)

Scientists have produced a new type of superconductor by manipulating graphene, the study of which led to a Nobel Prize. A team of Chinese scientists have manipulated the charge and the degree of freedom, known as spin, of electrons and their associated magnetic properties in a single-layer carbon material called graphene, making it suitable for applications involving superconductivity, a quantum mechanical phenomenon in which electrons travel in a material with no electrical resistance.
The authors investigated the means of exploiting a superconducting graphene flake. They looked at how unconventional electron pairing - known as d-wave pairing symmetries - would affect coherent subatomic - i.e., quantum - level transport within the material. In particular, they focused on electrical conductance in a system called a spin valve, which consists of two conducting magnetic materials, including a normal metal and graphene superconductor, the electrical resistance of which alternates from so-called giant magnetic resistance to none, depending on the alignment of its magnetic layers.
By reversing the magnetisation direction of one of the layers of the valve, researchers found that it is possible to achieve a spin-switch effect, whereby a normal current is converted into a superconducting current.
The authors hope that their theoretical results can provide the basis to design a spin-switch electron device able to operate at so-called high temperature, where liquid nitrogen can be used as a refrigerant.
Spin-switch effect in a graphene d-wave superconductor spin valve
C. Bai, J.T. Wang, H.X. Tang and Y.L. Yang, Eur. Phys. J. B 84, 83 (2011)
[Abstract]
Cosmology in a Petri dish (Vol. 43 No. 2)
Methods to study the formation of our universe are used to understand long-range interactions between particles at the micrometric scale. In the article summarised here, it it is shown that micron-size particles trapped at fluid interfaces exhibit a collective dynamic that is subject to seemingly unrelated governing laws smoothly transitioning from long-ranged cosmological-style gravitational attraction down to short-range attractive and repulsive forces.
The authors used so-called colloidal particles that are larger than molecules but too small to be observed with the naked eye, which are adsorbed at the interface between two fluids and assembled into a monolayer. This constitutes a 2D model in which particles larger than a micron deform the interface through their own weight and generate an effective long-range attraction which looks like gravitation in 2D, and thus assemble in clusters.
To model long-range forces between particles, numerical simulations based on random movement of particles, known as Brownian dynamics, have been used. It takes advantage of the formal analogy between so-called capillary attraction - the long-ranged interaction through interface deformation - and gravitational attraction.
It is also found that this long-range interaction no longer matters beyond a certain length determined by the properties of both the particles and the interface, and short-range forces come into play. This means that for systems exceeding this length, particles first tend to self-assemble into several clusters which eventually merge into a single, large cluster.
The study of monolayer aggregates of micron-size colloids are used in nanotechnology applications.
Collective dynamics of colloids at fluid interfaces
J. Bleibel, A. Dominguez, M. Oettel and S. Dietrich, Eur. Phys. J. E, 34, 125 (2011)
[Abstract]
Analysis of dynamic nano-scale interactions (Vol. 43 No. 2)

The understanding of energy transfer in the nanoscale could lead to a myriad of novel applications from the development of highly energy-efficient systems to the fabrication of materials that can either release or absorb large amounts of energy in an impact.
In dynamic atomic force microscopy (AFM), a microsized cantilever, with a sharp tip at its end, vibrates in the proximity of a surface and its dynamics are monitored to reveal chemical and mechanical properties about the sample. The effective volume affecting the dynamics of the cantilever is small enough that atomic and molecular processes can be probed. In particular, it is the phase lag between the drive and the oscillation amplitude of the cantilever that has been shown to provide information about dissipative processes. This link between energy dissipation and phase lag has attracted much attention since it is clear that it allows experimentally probing energy transfer in the nanoscale thus opening the door to fundamental discoveries in the field.
In this work, a direct and experimentally plausible method to disentangle two fundamental dissipative mechanisms, namely viscous and hysteretic processes, is provided. It further shows that the versatility of the AFM allows probing short- and long-range dissipative interactions. Short-range dissipation is identified with processes occurring at the interface where mechanical contact occurs whereas long-range dissipation is related to the chemical affinity between the interacting surfaces. Experimentally, we use a carbon nanotube grown on a quartz surface as a model system and show how both visco-elastic and hysteretic processes can be easily disentangled from the phase signal. The identification of dissipative mechanisms in samples of current interest should prove useful for the rapid advancement of nanotechnology.
Disentangling viscous and hysteretic components in dynamic nanoscale interactions
K.R. Gadelrab, S. Santos, T. Souier and M. Chiesa, J. of Phys. D: Appl. Phys. 45, 012002 (2012)
[Abstract]
Strong field ionization yields in linear and asymmetric top molecules (Vol. 43 No. 2)

Ionization is a ubiquitous process when atoms and molecules are exposed to strong laser fields from femtosecond (fs) laser pulses. For molecules the understanding of how the direction of the electric field from the laser, with respect to the molecular orientation, influences ionization represents a particularly important point. Here we present a joint experimental and theoretical study on the orientational dependence of ionization of carbonyl sulphide (OCS), benzonitrile and naphthalene molecules by an intense linearly polarized laser pulse. Experimentally this is achieved by fixing the spatial orientation, by laser-based methods, of the molecules prior to the ionization event. For naphthalene and benzonitrile, the orientational dependence of the ionization yield agrees well with the calculated results, in particular, we observe that ionization is maximized when the probe laser is polarized along the most polarizable molecular axis. For OCS maximum ionization yield occurs when the probe is perpendicular to the internuclear axis, which is at odds with the calculated results.
Our findings suggest that the orientational dependence of the total ionization yields in linearly polarized fields is not only determined by the ionization rate but re-scattering of the released electron on the parent ion, left behind, must also be accounted for. This possesses new challenges for future theoretical modeling.
Orientation-dependent ionization yields from strong-field ionization of fixed-in-space linear and asymmetric top molecules
J. L. Hansen, L. Holmegaard, J. H. Nielsen, H. Stapelfeldt, D. Dimitrovski and L. B. Madsen, J. Phys. B: At. Mol. Opt. Phys. 45, 015101 (2012)
[Abstract]
"Spooky action at distance" in particle physics?! (Vol. 43 No. 2)

The article summarised here presents the first conclusive test to better understand high-energy particles correlations: A proposal is devised for the first conclusive experimental test of a phenomenon known as 'Bell's nonlocality'. It is designed to reveal correlations that are stronger than any classical correlations, and to do so between high-energy particles that do not consist of ordinary matter and light. These results are relevant to the so-called 'CP violation' principle, which is used to explain the dominance of matter over antimatter.
In 1964, John Bell found that so-called local realistic hidden parameter theories imply that the relations between these high-energy particles correlations could be experimentally tested through so-called Bell tests.
In this study, the authors have succeeded in devising a new Bell test taking into account the decay property of high-energy particles systems, called kaon-antikaon systems, while ensuring that the test is conclusive - a goal that has never before been achieved - and simultaneously guaranteeing its experimental testability. Experimental testing requires equipment such as the KLOE detector at the accelerator facility DAPHNE in Italy.
Revealing "spooky action at distance" for kaon-antikaon pairs has fundamental implications for our understanding of such particles' correlations and could ultimately allow us to determine whether symmetries in particle physics and manifestations of particle correlations are linked.
Revealing Bell's Nonlocality for Unstable Systems in High Energy Physics
B. C. Hiesmayr, A. Di Domenico, C. Curceanu, A. Gabriel, M. Huber, J-Å. Larsson and P. Moskal, Eur. Phys. J. C, 72, 1856 (2012)
[Abstract]
Exploring charge transport in nitride heterostructures (Vol. 43 No. 2)
One of the most fundamental properties of nitride semiconductors is the presence of strong electronic polarization fields due to its wurtzite crystal structure. A variety of electronic and optoelectronic devices use or are adversely affected by the presence of polarization fields and charges at heterojunctions. For example, high GaN electron mobility transistors (HEMTs) use the high-density 2-dimensional electron gases induced by polarization discontinuity as the conductive channel. Optical devices such as light emitting diodes (LEDs) and lasers have to be designed by taking the presence of high polarization fields and quantum-confined Stark-effect into account. As investigations of non-polar and semi-polar orientations of GaN heterostructures mature for device applications, a comparative study of charge transport properties for various polar orientations in III-Nitride semiconductors is especially timely.
This article presents such a comparative study. Carrier transport is anisotropic in semi-polar and non-polar nitrides compared to the polar orientations. New phenomena such as interface charge scattering due to polarization discontinuity emerge for non-polar heterostructures. It is shown that for quantum well LEDs, the nitrogen-polar structure has the best carrier injection properties into the wells, while the non-polar structure has the highest electron-hole wave function overlap, enhancing the efficiency of light emission. Thus, depending on the dopant activation energies, polarization can help improve LED performance if the direction is chosen carefully. The study also reveals that by vertically injecting the high-energy electrons from AlGaN into GaN, the negative mass region of the GaN band structure can be accessed leading to current instabilities and current oscillation in the time domain. This process can be a recipe for generating ac power at high frequencies. This study will help to design high-mobility enhancement-mode transistors, efficient quantum well LEDs and possibly open up routes to nitride-based terahertz generation and detection.
Charge transport in non-polar and semi-polar III-V nitride heterostructures
A. Konar, A. Verma, Tian Fang, Pei Zhao, R. Jana and D. Jena, Semicond. Sci. Technol. 27, 024018 (2012)
[Abstract]
Atom distributions in a low-pressure He-Xe discharge (Vol. 43 No. 2)

Low-pressure glow discharges in mixtures of xenon with rare gases are used for lighting purposes in mercury-free fluorescent lamps. Over the last decade investigations have been carried out on their output characteristics in dependence on parameters such as mixture composition, pressure, discharge current and operation mode. However, the lifetime of these types of fluorescent lamps is limited by the performance of the electrodes and their interaction with the surrounding plasma. When using a flat oxide cathode a hot spot and a plasma can be generated. This spot mode changes the plasma-cathode interaction and prolongs the lifetime. The source of the plasma is localized in a very narrow region, but the plasma size is much bigger than the hot spot size. Outside of the cathode spot the plasma is sustained by transport of excited species, namely xenon atoms in the metastable and resonance states. Therefore, the discharge in spot mode can be used for investigations of the role of various transport mechanisms.
In the present work the method of laser atom absorption spectroscopy was used to measure the spatial distribution of excited xenon atoms in radial and axial dimensions. The analysis of the experimental results have been performed by means of numerical model which comprises the solution of the transport equations for metastable and the resonance atoms. Various approximations for the description of particle and radiation transport have been considered. It has been shown that the expansion of the plasma is mainly caused by transport of the resonance radiation. Suggested numerical method can be further used for precise description of density distribution of excited atoms and predictions of output characteristics of luminescent lamps.
Spatial distribution of metastable and resonance atoms in a low-pressure He-Xe discharge in spot mode
Yu. B. Golubovskii, S. Gorchakov, H. Lange, A. Timofeev, D. Uhrlandt and J. Winter, J. Phys. D: Appl. Phys. 45, 055205 (2012)
[Abstract]
Pre-suffused surfaces make them slippery (Vol. 43 No. 2)

Liquid drops most often stick to solids, which contributes to degrade these solids and affects their transparency. Slip can be induced by coating solids with hydrophobic textures: then, liquids only contact the texture tips, which dramatically decrease adhesion. On these super-hydrophobic materials, water nicely recovers the mobility expected from its low viscosity.
Another way to make liquids mobile was proposed in this letter and by Wang et al. (Nature 2011). It uses textures in a oleophilic situation: a solid coated with posts contacting oil can be spontaneously invaded by a film of this oil, the network of pillars acting as a kind of porous medium. At the texture scale (10 µm, typically), gravity is negligible compared to surface forces, so that the film gets trapped by the pillars, even when tilted. If now a drop contacts this substrate, it lands on a substrate mostly wet, and pinning can be strongly reduced. As an example, a coffee drop evaporating on a standard substrate leaves behind a coffee stain, primarily arising from the ability of the liquid to stick, while the coffee powder gets localized on these new slippery materials - making it easy to remove afterwards.
The condition for achieving these "floating" states was explored: the pre-suffused oil must wet the substrate with air above, but also with water (or another oil) above. Apart from its potential applications, this system is one of the very first explored where four phases (instead of three, in classical wetting) meet. It also has the interesting capacity to dissolve incoming liquid contaminants, again taking advantage of the mostly-liquid nature of the substrate.
Slippery pre-suffused surfaces
A. Lafuma and D. Quéré, EPL, 96, 56001 (2011)
[Abstract]
Rydberg-hydrogen collisions with surfaces: detection of electrons (Vol. 43 No. 2)
In the Rydberg electronic states of atoms and molecules, one electron has been excited into a distant orbital and is very weakly bound, giving exotic physical and chemical characteristics. The Rydberg orbital can be easily polarized using weak electric fields, and when the Rydberg state is near a surface the electron distribution may be selected to be oriented towards, or away from, the surface (see figure). The van der Waals force between the atom and surface strongly perturbs the Rydberg electron, and at a distance of ~4 times the mean orbital radius the electron may transfer into the surface conduction band. The precise distance at which electron-transfer occurs is controllable by populating Rydberg states with different principal quantum numbers or different surface orientations.
In previous experiments this process was studied by detecting positive ions formed as the Rydberg species ionizes. In the present work electrons have been detected experimentally for the first time from the surface-interaction of both H atoms and H2 molecules in Rydberg states in the presence of a weak electric field normal to the surface. The electron flux away from the surface occurs because the surface interaction lowers the potential energy barrier on the vacuum-facing side of the atom allowing electron escape in that direction. In addition to the novel physics of the process, an important implication of the electron extraction is that it allows the positive ions to impact with the surface under extremely low (thermal) energy conditions. The collision energy is dependent on the ionization distance, which is determined by the initial Rydberg state selected. This opens opportunities to exploring novel forms of thermal ion-surface physics with a range of surfaces and adlayers.
Detection of electrons in the surface ionization of H Rydberg atoms and H2 Rydberg molecules
E. A. McCormack, E. So, M. Dethlefsen, M. S. Ford and T. P. Softley, J. Phys. B: At. Mol. Opt. Phys. 45 (2012) 015204
[Abstract]
Largest ever gas mix caught in ultra-freeze trap (Vol. 43 No. 2)


The article summarised here leads to a better understanding of subatomic particles using a new cold-atom setup. The work make it easier to study atomic or subatomic-scale properties of the building blocks of matter (which also include protons, neutrons and electrons) known as fermions by slowing down the movement of a large quantity of gaseous atoms at ultra-low temperature.
Thanks to the laser cooling method for which Claude Cohen-Tannoudji, Steven Chu and William D. Phillips received the Nobel Prize in 1997, the authors succeeded in creating the largest Lithium 6 (6Li) and Potassium 40 (40K) gas mixture to date. The experimental approach involved confining gaseous atoms under ultra-high vacuum using electromagnetic forces, in an ultra-freeze trap of sorts.
This trap enabled them to load twice as many atoms than previous attempts at studying such gas mixtures, reaching a total on the order of a few billion atoms under study at a temperature of only a few hundred microKelvins (corresponding to a temperature near the absolute zero of roughly -273 °C).
Given that the results of this study significantly increased the number of gaseous atoms under study, it will facilitate future simulation of subatomic-scale phenomena in gases. In particular, it will enable future experiments in which the gas mixture is brought to a so-called degenerate state characterised by particles of different species with very strong interactions. Following international efforts to produce the conditions to study subatomic-scale properties of matter under the quantum simulation program, this could ultimately help scientists to understand quantum mechanical phenomena occurring in neutron stars and so-called many-body problems such as high-temperature superconductivity.
Large atom number dual-species magneto-optical trap for fermionic 6Li and 40K atoms
A. Ridinger, S. Chaudhuri, T. Salez, U. Eismann, D. Rio Fernandes, D. Wilkowski, F. Chevy and C. Salomon, Eur. Phys. J. D, 65, 1-2. (2011) - cold quantum matter special issue.
[Abstract]
Tailoring AlGaInAs quantum dot solar cells (Vol. 43 No. 2)

One of the most important challenges in seeking for competitive energy resources is to increase the efficiency of solar cells. The intermediate band solar cell promises highest efficiencies in a single gap device, since it allows for the absorption of below-bandgap photons via a subband located within the host material's bandgap. In order to generate an isolated subband, electronically coupled stacks of quantum dots (QDs) have been proposed. The built-in potential in the intrinsic region of the solar cell however unavoidably lifts the approximate degeneracy between the QD electron eigenenergies. Hence, the wave functions in adjacent layers would be electronically decoupled, resulting in carrier localization.
To avoid this effect and facilitate the formation of a QD-subband, the present work focuses on the integration of tailored quaternary AlGaInAs QDs. it deals with three major goals in quantum dot solar cell research: Increasing QD absorption by the fabrication of high areal density QDs, engineering the QD's bandgap to tailor their absorption range, and preserving the possibility to maintain an intermediate band despite the built-in potential being present in solar cell p-n-junctions. By a suitable choice of QD composition, shape and barrier thickness they show by simulations routes toward QD-IBSC designs, whereas they experimentally demonstrate a widened spectral absorption range.
AlGaInAs quantum dot solar cells: tailoring quantum dots for intermediate band formation
C. Schneider, S. Kremling, N. V. Tarakina, T. Braun, M. Adams, M. Lermer, S. Reitzenstein, L. Worschech, M. Kamp, S. Höfling and A. Forchel, Semicond. Sci. Technol. 27 032002 (2012)
[Abstract]
Speed of sound in a complex plasma under microgravity (Vol. 43 No. 2)
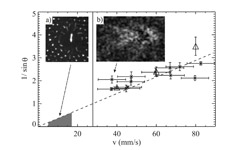
Complex plasma experiments under microgravity conditions in the International Space Station ISS, complementing research on Earth, are very important for the progress in this field. Complex plasmas consist of micrometre-sized highly charged particles ("microparticles") embedded in an ionized gas ("plasma"). The microparticle ensemble resembles a classical system of interacting atoms. This system can form all of the classic phases, i.e., crystalline, liquid and gaseous. It can also support the propagation of sound waves, solitons and shock waves.
In experiments carried out on Earth, gravity pulls the microparticles downwards resulting in two-dimensional structures. Under microgravity conditions it is possible to investigate big, three-dimensional systems. The PK-3 Plus laboratory provides ideal conditions for such experiments. It is installed in the Russian segment of the ISS and has been used repeatedly over the last 6 years.
We performed experiments to measure the speed of sound during two missions with cosmonauts Volkov and Skvortsov. In Figs. (a) and (b) we show how a bigger "probe" particle penetrated a cloud of smaller microparticles. At a speed several times faster than the speed of sound it excited a Mach cone. A double cone structure is discernible in this "atomistic" system. While moving through the cloud, the probe particle is decelerated to subsonic speeds. By measuring the Mach cone angle at several probe particle velocities, we determined the Mach relation. This allows us to directly measure the speed of sound and to infer the microparticle charge. In addition, the experimental results agree well with a 3D molecular-dynamics simulation, demonstrating in particular a double Mach cone structure.
Direct measurement of the speed of sound in a complex plasma under microgravity conditions
M. Schwabe, K. Jiang, S. Zhdanov, T. Hagl, P. Huber, A. V. Ivlev, A. M. Lipaev, V. I. Molotkov, V. N. Naumkin, K. R. Sütterlin, H. M. Thomas, V. E. Fortov, G. E. Morfill, A. Skvortsov and S. Volkov, EPL, 96, 55001 (2011)
[Abstract]
How massless electrons tunnel through graphene (Vol. 43 No. 2)
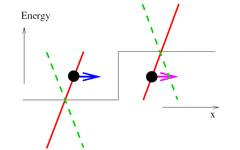
Electrons moving in graphene behave in an unusual way, as demonstrated by 2010 Nobel Prize laureates for physics A. Geim and K. Novoselov, who performed transport experiments on this one-carbon-atom-thick material. The present review explores the theoretical and experimental results to date of electrons tunnelling through energy barriers in graphene.
What could partly explain graphene's properties is that electrons travelling inside the material behave as if they were massless. Their behaviour is described by the so-called Dirac equation, which is normally used for high-energy particles such as neutrinos in vacuum moving at a velocity 300 times greater than that of electrons, nearing the speed of light.
In this review, the authors focus on the tunnelling effect occurring when Dirac electrons found in graphene are transmitted through different types of energy barriers. Contrary to the laws of classical mechanics, which govern larger scale particles that cannot cross energy barriers, electron tunnelling is possible in quantum mechanics - though only under restricted conditions, depending on the width and energy height of the barrier.
However, the Dirac electrons found in graphene can tunnel through energy barriers regardless of their width and energy height; a phenomenon called Klein tunnelling, described theoretically for 3D massive Dirac electrons by the Swedish physicist Oskar Klein in 1929. Graphene was the first material in which Klein tunnelling was observed experimentally, as massive Dirac electrons required energy barriers too large to be observed.
Klein tunnelling in graphene: optics with massless electrons
P.E. Allain and J.N. Fuchs, Eur. Phys. J. B 83, 301 (2011)
[Abstract]